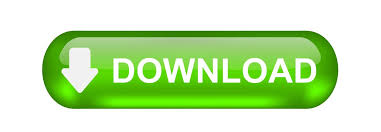
Given the differences in half-lives for various nuclides, there are optimum times to count an activated sample. Hence, a correction that must be made, when U is present, is for the amount of a nuclide produced by the fission process. When subjected to a thermal neutron flux 235U atoms in a sample will undergo fission producing a variety of fission products, some of which are nuclides of interest. Where A is the activity at any time t, A o is the initial activity, λ is the decay constant and t is time. The standard form of the radioactivity decay correction is Each radioactive nuclide is also decaying during the counting interval and corrections must be made for this decay.
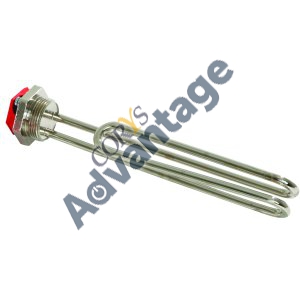
Because there can be thousands to hundreds of thousands of gamma ray interactions per second with the detector, an important criteria in system design is the response time of the detector to gamma ray interactions (as measured by the dead time). The number of electrons (current) is related to the energy of the gamma ray. Gamma rays passing through the detector generate free-electrons. Where t 1/2 is the half-life and λ is the decay constant.Īfter the sample has been activated, the resulting gamma ray energies and intensities are determined using a solid-state detector (usually Ge).

This can be done using the following equation It is sometimes useful to convert from half-life to decay constant. Because the neutron flux is not constant, the total flux (called fluence) received by each sample must be determined using an internal or external fluence monitor. The optimum irradiation time depends on the type of sample and the elements of interest. What this means is that the rate of production and decay is now in equilibrium and further irradiation will not lead to an increase in activity. If the irradiation time is much longer than the half-life of the nuclide, saturation is achieved. Note that for any particular radioactive nuclide radioactive decay is occurring during irradiation, hence the total activity is determined by the rate of production minus the rate of decay. From this equation we can see that the total activity for a particular nuclide is a function of the activation cross-section, the neutron flux, the number of parent atoms, and the irradiation time. Where A t = the activity in number of decays per unit time, σ act = the activation cross-section, φ = the neutron flux (usually given in number of neutrons cm -2 s -1), N = the number of parent atoms, λ = the decay constant (number of decays per unit time), and t = the irradiation time. The activity for a particular radionuclide, at any time t during an irradiation, can be calculated from the following equation For routine neutron activation analysis we are generally looking at nuclides that are activated by thermal neutrons.
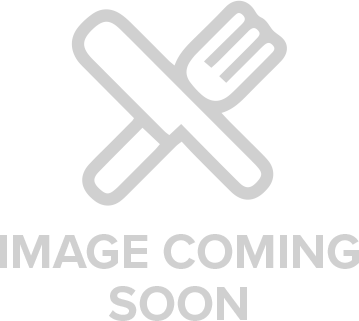
/is2.ecplaza.com/ecplaza2/products/c/cd/cdf/1557602938/fiscal-cash-register.jpg)
Some nuclides have greater capture cross-sections for higher energy neutrons (epithermal neutrons). For many nuclides, the capture cross-section is greatest for low energy neutrons (referred to as thermal neutrons). This probability is referred to as the capture cross-section, and each nuclide has its own neutron energy-capture cross-section relationship. The probability of a neutron interacting with a nucleus is a function of the neutron energy. The gamma rays emitted during the decay of the 59Fe nucleus have energies of 142.4, 1099.2, and 1291.6 KeV, and these gamma ray energies are characteristic for this nuclide. Details 58Fe is a stable isotope of iron while 59Fe is a radioactive isotope.
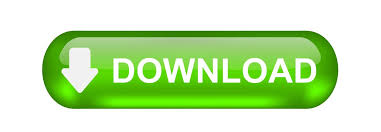